CO2 Capture From Power Plants
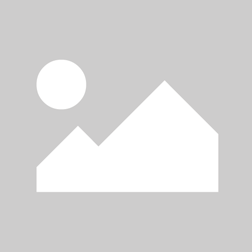
This training reviews the application of CO2 capture from power plants and how it will contribute to a clean and stable electric grid. This training is part of a series of trainings on achieving a clean electric grid and some of the main technologies that will get us there.
Carbon capture, utilization, and storage (CCUS) background
Anytime a carbon-containing substance is burned, it produces carbon dioxide (CO2), the principal greenhouse gas driving climate change. Finding ways to separate out that CO2 before it exhausts into the atmosphere and then immobilize it somewhere would help slow down global warming. This is broadly termed carbon capture, utilization, and storage (CCUS).
Methods to capture CO2 in various solvents have been around for more than 100 years, so applying those methods to remove CO2 emissions from power plants is a relatively straightforward idea, if challenging in practice.
The second step — extracting the captured CO2 from the solvent and storing it safely — is a newer challenge. One secure option for this step is called geological sequestration, where the gas is pumped deep underground into geological formations where it stays put or even becomes “mineralized,” or chemically bonded to certain types of rock.
Image: Environmental Defense Fund
Although CO2 capture has been practiced in certain industries since the 1920s, there was no economic incentive for electric utilities to capture and sequester it until the 45Q tax credit was established in 2008 and then further boosted via the Inflation Reduction Act in 2022. That policy will pay a facility $85 per ton of sequestered CO2 for 5 years after startup (12 years for not-for-profit entities). The facility must capture at least 75% of their CO2 emissions to qualify, which is technically feasible now that the technology has advanced to the point that capture efficiency could approach 100%, although so far most projects have underperformed in their CO2 capture efforts.
CO2 sequestration in underground geological formations offers by far the greatest potential for permanent storage; the International Energy Agency estimates a potential capacity that could store between 215 and 1490 years of current CO2 emissions, and if storage in basalt formations proves to be feasible at large scale, that potential would grow a thousandfold.
There are also various ways to utilize (this is the “U” in CCUS) the captured carbon in products, such as in chemicals, building materials, or polymers, that would keep it out of the atmosphere. Utilization options may be economically attractive in some cases, but the total potential is only a few percent as much as geological storage. Also, some of the products typically cited as “utilization” options (e.g., fuels or chemicals that end up releasing CO2) do not permanently sequester the carbon.
How CCUS supports a clean and stable electric grid
Power plants are responsible for 25% of our nation’s CO2 emissions, and most of it pours out of smokestacks. But even in the face of climate urgency, some of those power plants will still need to keep operating for some time to ensure grid stability — even a brief disruption in voltage or frequency can damage equipment and cause utility customers to lose power. Furthermore, as the contribution of variable renewables grows, dispatchable generators are needed to back them up.
Retrofitting existing power plants with CCUS systems can potentially provide clean firm power for the grid with minimal disruption. It also facilitates efforts to ensure a just transition for fossil-based utility workers. Even under the most optimistic scenarios for renewable deployment, it will still take decades to fully make that transition in an orderly way. With sufficient economic incentives, CCS technology can be promptly deployed to existing fossil plants — natural gas in particular.
CCUS combined with bioenergy can also function as a carbon-negative approach via the bioenergy with carbon capture and storage (BECCS) route. The IPCC has forecast that carbon-negative technologies like BECCS will be needed in order to achieve net-zero conditions by mid-century. The promise of net CO2 removal from the atmosphere is an advantage that can’t be offered by any other power-generating technology.
Issues with CCUS
Many groups fear that actively supporting CCUS will divert resources away from other clean energy options and lead to technology “lock-in” as new natural gas plants are built and commissioned. Upstream environmental damage and GHG emissions from fossil fuel extraction and distribution, including methane leakage from natural gas infrastructure, would persist. This is of particular concern to environmental justice advocates.
A related concern is the need to expand the existing network of CO2 pipelines. This has already stirred up local opposition to pipelines linking midwestern ethanol refineries to sequestration sites.
As for BECCS, there are two main downsides. First, there is the threat of conflict over land use if growing biomass for energy leads to “energy plantations” that could erode biodiversity, harm watersheds, reduce the amount of arable land available for food production, and lead to excessive use of fertilizers and pesticides. In theory, this could be avoided under strict guidelines, but there is no guarantee it won’t get out of hand. Second, biomass is a relatively inefficient combustion fuel due to its high oxygen content, high moisture content, and low density. This leads to lower energy efficiency compared to more concentrated fossil fuels, making it more economically challenging for power generation.
How much could CCUS contribute to a clean and stable electric grid?
The Princeton Net-Zero Report describes five distinct net-zero pathways for the U.S. that would depend on a combination of political and market decisions. All of the scenarios assume that some CO2 — 0.7 to 1.8 billion metric tons — is captured, mainly from natural gas and/or biomass. Four of the five presume >82% of it would end up underground, while in the fifth scenario, all of the captured CO2 would be chemically converted to zero-carbon synthetic fuels (also termed electrofuels), including synthetic methane, diesel, gasoline, and jet fuel.
In 2050, according to the Princeton analysis, the amount of generated power that would be directly equipped with CCUS ranges from 40 to 1610 TWh, which is 0.3% to 19% of total U.S. demand. In scenarios where the grid is less dependent on CCUS, other sectors like transportation and industry will be more dependent on it. The large range depends on a variety of factors, such as how much total electricity is needed, which in turn depends on how much each sector of the economy electrifies, CCUS regulations and policy, and how much electricity demand is met by other technologies.
Other FAQs
How does CCUS work with different fuels?
The process, cost, and environmental implications are different for different fuels. The diagram below helps to show why.
First of all, exhaust gases have to be cleaned up in certain ways before CO2 can be removed, which affects cost and complexity. Second, the climate impact of CO2 emissions is different for fossil fuels than for bio-derived fuels.
Start with coal, which provides 20% of electricity in the U.S. and 26% worldwide. Coal presents problems for CO2 capture because of high levels of nitrogen oxides (NOx), particulate solids, and sulfur gases that need to be removed. The role of coal in power generation is already declining fast anyway, so it’s unlikely we will see significant deployment of CCS at coal plants.
Next is natural gas, which provides 40% of U.S. electricity and 22% worldwide. Because nitrogen oxide levels are lower and it’s not saddled with the need to remove particulates and sulfur, it is the most likely power plant fuel to see a growth in CCS add-ons. Some new natural gas power plants are even designed from the ground up for high energy efficiency and 99% CO2 capture.
Last but not least is biomass. Although it currently only provides 1.3% of electricity in the U.S. and 1.9% worldwide, biomass offers the potential to generate carbon-negative power when combined with CCS. The range of applications is likely to be limited due the low energy density and high moisture content of biomass, as well as ecosystem and land use restrictions. Consequently, deployment of BECCS for power generation will probably begin where centralized supplies of waste biomass are available, such as in or near paper, lumber, and sugar mills.
Is CCUS safe and permanent?
Questions have arisen about the permanence and safety of underground CO2 sequestration. Carbon dioxide is not toxic per se, but it is a potential asphyxiant and, being heavier than air, could create a hazard to people or animals if it leaked. It would be imperative for agencies to impose strict regulations to minimize hazards and require adequate monitoring for leaks from pipelines and storage sites.
The question is also raised whether CO2 sequestered underground will truly benefit the climate if it doesn’t stay there. Current practice is to pump it down into porous sedimentary rock reservoirs far below a non-permeable “caprock.” Experience to date shows good results, as long as best practices are followed. A better way to ensure permanence is to put the CO2 where it can combine chemically with existing geology, a process called mineralization. Storage of captured CO2 in basalt formations that behave this way has already shown great promise, and the worldwide potential is vast (>60,000 GtCO2).
What about captured CO2 used for enhanced oil recovery?
When oil wells start to run dry, CO2 is routinely used to force out more of the crude oil – a practice that is called enhanced oil recovery (EOR). In the past, the required CO2 was obtained from naturally occurring underground reservoirs, but CO2 from industrial CCS operations has increasingly been sold for EOR purposes, in order to offset the cost of the CCS process. This has raised objections from environmental groups, which claim that the climate benefit of the CO2 sequestration is lost because the recovered oil would result in more emissions.
However, oil consumption responds to market prices, so oil produced by EOR displaces oil extracted by other methods and so doesn’t necessarily result in more emissions, and the CO2 still remains sequestered. Besides, getting more oil from existing wells may conceivably result in fewer new wells being drilled, and thus less environmental impact. Even so, CO2 captured and sold for EOR receives a lower tax credit ($60 per ton) than CO2 captured for other purposes ($85 per ton).
Is CCUS economical?
For an electricity supplier, simply letting CO2 go up the stack has zero cost, which is why carbon capture has not been used except in a few demonstration plants. But climate urgency compels us to change that. For U.S. power generation, the added cost of CCUS, including transport and storage, ranges widely from $60 to $330 per ton of CO2. For comparison, the estimated CO2 cost for other clean firm sources like geothermal and nuclear also range widely, from $0 to $230 per ton, depending on source.
The most straightforward way to internalize this cost is in the context of carbon pricing, where power plants that send CO2 to a sequestration site would get a CO2 rebate equal to the carbon price they originally paid. This is what CCL has consistently proposed, and continues to work on. But in the absence of that policy, the 45Q tax credit now appears to be sufficient to drive CCUS investment, at least for natural gas. Utilization options will also figure into the economics in cases where the product made from CO2 has a market value.
The cost impact on grid power can be expressed as the levelized cost of electricity (LCOE) in dollars per megawatt-hour ($/MWh). For natural gas combined cycle, the LCOE is estimated at $45-$74/MWh. Adding a CO2 capture unit, per an MIT study, would raise that by $33-$49/MWh, resulting in an unsubsidized cost of $78-$123/MWh, but with the 45Q tax credit, that would drop to $48-$93/MWh. For comparison, the LCOE of other clean firm power comes in at $56-$93/MWh for geothermal, $29-$204/MWh for nuclear, and $64/MWh for hydropower.
Does CCUS work for industries other than power generation?
Outside the power sector, there are numerous industries that are well-suited to CCUS implementation. These include steel, cement, fertilizer, and chemicals. For BECCS in particular, ethanol, other biofuels, and cement already use biomass and thus are well-suited to full or partial BECCS.
However, since this training is focused on the electric power sector, it won’t go into more detail on industrial CCUS applications.